Regular Articles
Vol. 21, No. 3, pp. 27–33, Mar. 2023. https://doi.org/10.53829/ntr202303ra1
Over 100-Tbit/s Ultra-wideband Wavelength Division Multiplexed Transmission Technologies for Future Optical Transport Network Systems
Fukutaro Hamaoka, Kohei Saito, Akira Masuda,
Hiroki Taniguchi, Takeo Sasai, Masanori Nakamura,
Takayuki Kobayashi, and Yoshiaki Kisaka
Abstract
This article reviews trends in ultra-wideband wavelength-division multiplexing (WDM) transmission techniques for expanding the capacity of optical transmission systems. It also presents NTT’s recent research and development results on ultra-wideband WDM transmission for over 100-Tbit/s transmission capacities in a triple-band (S, C, and L bands) WDM configuration.
Keywords: digital coherent, wavelength division multiplexing, digital signal processing
1. Introduction
To cope with the rapid increase in communication traffic, it is necessary to increase the transmission capacity per optical fiber while reducing the cost per bit of optical transmission systems. Innovative technologies, such as wavelength-division multiplexing (WDM) transmission with optical amplifiers and digital coherent technology with digital signal processing (DSP) [1], have continuously been expanding the system capacities for optical transmission. DSP application-specific integrated circuits (ASICs) for digital coherent technology support multi-rate and multi-modulation formats to meet the demand for multiple applications: long-haul, metro, and short-reach (particularly datacenter interconnects) networks. For example, a DSP-ASIC can execute ~32-GBaud polarization-division multiplexed (PDM) quadrature amplitude modulation (QAM) formats with modulation orders from 4 to 16 for from 100 to 200 Gbit/s/carrier [2]. Up to 600-Gbit/s/carrier with 64QAM transmission experiments have also been reported with a real-time transponder including a 64-GBaud-class DSP-ASIC [3]. NTT has recently developed a cutting-edge DSP and an optical device supporting 1.2 Tbit/s/carrier with 140-GBuad-class coded 64QAM for digital coherent systems [4].
To further increase system capacity, expanding the WDM bandwidth is effective along with high spectral efficiency (SE) signals with digital coherent technology using high-order QAM formats. A transmission of 102.3-Tbit/s with PDM-64QAM signals has been demonstrated under the 11.2-THz dual-band (C and L bands) WDM condition with an SE of 9.1 bit/s/Hz [5]. NTT has also successfully demonstrated the first ever greater than 150-Tbit/s transmission with 272-channel PDM-128QAM signals under the 13.6-THz triple-band (S, C, and L bands) WDM condition with an SE of 11.05 bit/s/Hz [6]. Both experiments were conducted using the offline evaluation technique in which DSP is carried out on workstations for transmitted and captured received signals. The offline evaluation enables detailed signal analysis of DSP methods toward ASIC implementation.
In this article, we present real-time triple-band transmission with a WDM bandwidth of 16.95 THz using DSP-ASIC-integrated optical transponders. We transmitted total capacities of 102.7 and 112.8 Tbit/s using 226-channel WDM signals with 400-Gbit/s PDM-16QAM and 500-Gbit/s PDM-32QAM [7]. As can be seen in Fig. 1, we achieved, for the first time, over 100-Tbit/s capacity in real-time transmission experiments.
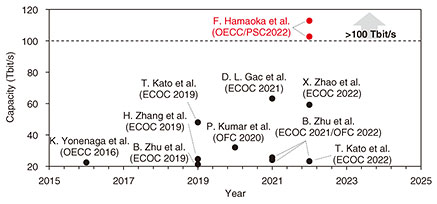
Fig. 1. Trends in capacity growth in digital coherent experiments for real-time transmission with DSP-ASIC-integrated optical transponders.
2. Experimental setup for ultra-wideband WDM transmission using DSP-ASIC-integrated optical transponders
Figure 2 shows the setup for triple-band WDM transmission experiments with real-time optical transponders. We used three real-time optical transponders having DSP-ASICs based on 16-nm complementary metal oxide semiconductor (CMOS) technology [3, 8]. The transponders were also implemented driver (DRV) amplifiers, a lithium niobate in-phase and quadrature modulator (LN-IQM), and a high-bandwidth intradyne coherent receiver (HB-ICR). Signal and local oscillator (LO) laser diodes (LD) sources were external cavity lasers for the S band and integrable tunable laser assemblies for the C and L bands. The modulation formats of the main signal were 67-GBaud PDM-16QAM with a net rate of 400 Gbit/s and 66-GBaud PDM-32QAM with a net rate of 500 Gbit/s, which were generated in the optical transponder for each WDM band. The carrier frequency of the main signal was set to 1467.233–1524.304 nm in the S band, 1530.529–1567.133 nm in the C band, and 1570.005–1607.897 nm in the L band.
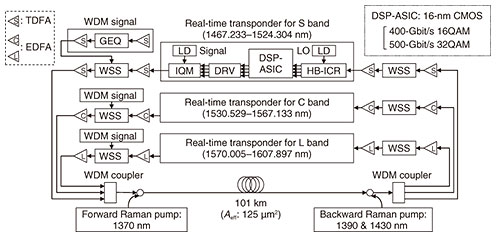
Fig. 2. Experimental setup for triple-band WDM transmission with DSP-ASIC-integrated real-time optical transponders.
The WDM signals in the S, C, and L bands were generated using amplified spontaneous emissions from the thulium-doped fiber amplifiers (TDFAs) and erbium-doped fiber amplifiers (EDFAs). The power level of the WDM signal was equaled using a gain equalizer (GEQ) on the basis of flexible-grid wavelength selective switches (WSSs) with liquid crystal on silicon (LCOS). TDFAs for the S band and EDFAs for the C and L bands were used in this experiment. The main signal from the real-time optical transponder and WDM signal for each band were multiplexed in a LCOS-based flexible-grid WSS. The WDM grid in this experiment was set to 75 GHz. The WDM signals in the S, C, and L bands were then multiplexed in a WDM coupler with a total bandwidth of 16.95 THz (7.725, 4.650, 4.575 in the S, C, and L bands, respectively), as shown in Fig. 3. The total number of WDM signals was 226 channels (103, 62, and 61 channels in the S, C, and L bands, respectively). The triple-band WDM signal was transmitted through the transmission line of a 101-km large-core low-loss fiber, which is compliant with ITU-T (International Telecommunication Union - Telecommunication Standardization Sector) G.654.E, with an effective area (Aeff) of 125 μm2. We used a forward (FW)-pumped distributed Raman amplifier (DRA) at a wavelength of 1370 nm and a backward (BW)-pumped DRA at 1390 and 1430 nm.
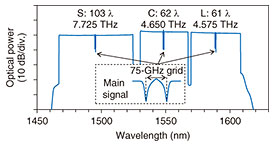
Fig. 3. WDM signal spectra of 16.95-THz bandwidth.
The triple-band WDM signal was divided into each S-, C-, and L-band WDM signal after 101-km optical fiber transmission. The WDM signals were then amplified using a TDFA for the S band and EDFAs for the C and L bands at the receiver side to compensate for the transmission losses. After filtering the main signal for each S, C, and L band using a LCOS-based flexible grid WSS, the signal was coherently detected with the HB-ICR with the optical LO. Finally, the received signal was equalized, demodulated, and decoded in the DSP-ASIC [3].
3. Experimental results of over 100-Tbit/s real-time transmission
In the ultra-wideband WDM configuration, inter-band stimulated Raman scattering (SRS) affects the WDM signals. A nonlinear interaction is caused by inter-band SRS in bands with a gap of around 100 nm for ultra-wideband transmission systems; inter-band SRS causes a signal power transition from S- to L-band signals in triple-band transmissions [6]. Therefore, we evaluated the effect of inter-band SRS with the 16.95-THz triple-band WDM configuration. The fiber loss at 101 km and transmission loss with inter-band SRS are shown in Fig. 4. The signal-power transition was mainly observed from the S band to the L band.
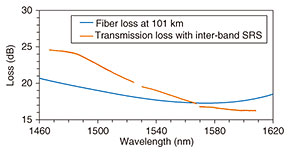
Fig. 4. 101-km fiber loss and transmission loss with inter-band SRS.
Figure 5 shows the experimental results in 101-km real-time triple-band WDM transmission. The FW- and BW-pumped DRA were applied for the transmission line to compensate for the excess power loss in the S band caused by the inter-band SRS. As Fig. 5(a) shows, the WDM signal power in the S band was drastically increased by the Raman amplifiers with sufficient Raman amplification gain. To evaluate the signal performance, we used the pre-forward error collection (FEC) quality (Q) margin, which is defined by the difference between the measured pre-FEC Q factor in the experiments and the required pre-FEC Q factor to achieve an error-free post-FEC bit error rate (BER). We observed that the pre-FEC Q margins of all measured 226-channel WDM signals showed more than zero, as shown in Fig. 5(b). We also confirmed that the post-FEC BER of all signals were error-free in this case. That is, in this setup, we achieved 112.8-Tbit/s (= 500 Gbit/s × 224 λ + 400 Gbit/s × 2 λ) transmission using real-time optical transponders.
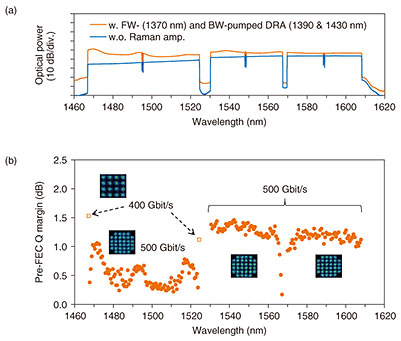
Fig. 5. Experimental results: (a) WDM optical spectra with and without FW- and BW-pumped DRA and (b) pre-FEC Q margin after 226-channel triple-band WDM transmission through 101-km fiber transmission with FW- and BW-pumped DRA.
The long-term signal performance under the 16.95-THz triple-band WDM condition was evaluated during transmission in 101-km fiber when applying the FW- and BW-pumped DRA. As shown in Fig. 6, we obtained stable signal-transmission performance at the center channels of S-, C-, and L-band signals with small pre-FEC Q-factor fluctuations of less than or equal to 0.036, 0.025, and 0.037 dB, respectively, within continuous measurements for 60 min. During the stability test, we also confirmed error-free operation after FEC decoding.
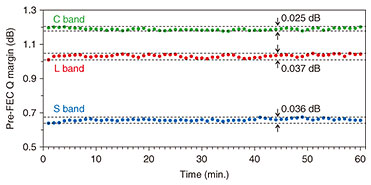
Fig. 6. Stability test for signal performance at center channels of S-, C-, and L-band signals with FW- and BW-pumped DRA during 101-km fiber transmission under 16.95-THz triple-band WDM condition within continuous measurements for 60 min.
4. Conclusion
We reviewed trends in ultra-wideband WDM transmission techniques to expand the capacity of optical transmission systems and NTT’s latest R&D in the field. With the combination of ultra-wideband WDM transmission and digital coherent technology using the state-of-the-art DSP-ASIC, we successfully demonstrated the first ever over 100-Tbit/s real-time transmission. This technology is promising for use in future optical transport network systems.
Acknowledgments
This work was supported by the Ministry of Internal Affairs and Communications, “The research and development project for the Tera-bit optical network technologies towards big data era” and “Research and Development of innovative optical network technology that bears new social infrastructure (Technological Theme I) (JP MI00316).”
References
[1] |
E. Yamazaki, S. Yamanaka, Y. Kisaka, T. Nakagawa, K. Murata, E. Yoshida, T. Sakano, M. Tomizawa, Y. Miyamoto, S. Matsuoka, J. Matsui, A. Shibayama, J. Abe, Y. Nakamura, H. Noguchi, K. Fukuchi, H. Onaka, K. Fukumitsu, K. Komaki, O. Takeuchi, Y. Sakamoto, H. Nakashima, T. Mizuochi, K. Kubo, Y. Miyata, H. Nishimoto, S. Hirano, and K. Onohara, “Fast Optical Channel Recovery in Field Demonstration of 100-Gbit/s Ethernet over OTN Using Real-time DSP,” Opt. Express, Vol. 19, No. 14, pp. 13179–13184, June 2011. |
[2] |
S. Okamoto, K. Yonenaga, K. Horikoshi, M. Yoshida, Y. Miyamoto, M. Tomizawa, T. Okamoto, H. Noguchi, J. Abe, J. Matsui, H. Nakashima, Y. Akiyama, T. Hoshida, H. Onaka, K. Sugihara, S. Kametani, K. Kubo, and T. Sugihara, “400 Gbit/s/ch Field Demonstration of Modulation Format Adaptation Based on Pilot-aided OSNR Estimation Using Real-time DSP,” IEICE Trans. Commun., Vol. E100-B, No. 10, pp. 1726–1733, Oct. 2017. |
[3] |
F. Hamaoka, T. Sasai, K. Saito, T. Kobayashi, A. Matsushita, M. Nakamura, H. Taniguchi, S. Kuwahara, H. Kawahara, T. Seki, J. Ozaki, Y. Ogiso, H. Maeda, Y. Kisaka, and M. Tomizawa, “Dual-carrier 1-Tb/s Transmission over Field-deployed G.654.E Fiber Link Using Real-time Transponder,” IEICE Trans. Commun., Vol. E103-B, No. 11, pp. 1183–1189, Nov. 2020. |
[4] |
NTT press release, “World’s largest capacity of 1.2 Tbit/s per wavelength by newly developed digital coherent signal processing circuit and optical device - For larger capacity, longer transmission distances, and lower power consumption in optical networks -,” Sept. 5, 2022.
https://group.ntt/en/newsrelease/2022/09/05/220905a.html |
[5] |
A. Sano, T. Kobayashi, S. Yamanaka, A. Matsuura, H. Kawakami, Y. Miyamoto, K. Ishihara, and H. Masuda, “102.3-Tb/s (224 × 548-Gb/s) C- and Extended L-band All-Raman Transmission over 240 km Using PDM-64QAM Single Carrier FDM with Digital Pilot Tone,” Proc. of the 35th Optical Fiber Communication Conference and Exposition and National Fiber Optic Engineers Conference (OFC/NFOEC 2012), Los Angeles, CA, USA, Paper PDP5C.3, Mar. 2012. |
[6] |
F. Hamaoka, M. Nakamura, S. Okamoto, K. Minoguchi, T. Sasai, A. Matsushita, E. Yamazaki, and Y. Kisaka, “Ultra-wideband WDM Transmission in S-, C-, and L-bands Using Signal Power Optimization Scheme,” J. Lightw. Technol., Vol. 37, No. 8, pp. 1764–1771, Apr. 2019. |
[7] |
F. Hamaoka, K. Saito, A. Masuda, H. Taniguchi, T. Sasai, M. Nakamura, T. Kobayashi, and Y. Kisaka, “112.8-Tb/s Real-time Transmission over 101 km in 16.95-THz Triple-band (S, C, and L Bands) WDM Configuration,” Proc. of the 27th OptoElectronics and Communications Conference and International Conference on Photonics in Switching and Computing (OECC/PSC 2022), Toyama, Japan, Paper PDP-A-3, July 2022. |
[8] |
O. Ishida, K. Takei, and E. Yamazaki, “Power Efficient DSP Implementation for 100G-and-beyond Multi-haul Coherent Fiber-optic Communications,” Proc. of the 39th Optical Fiber Communications Conference and Exhibition (OFC 2016), Anaheim, CA, USA, Paper W3G.3, Mar. 2016. |
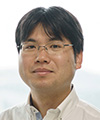 |
- Fukutaro Hamaoka
- Senior Research Engineer, NTT Network Innovation Laboratories.
He received a B.E., M.E., and Ph.D. in electrical engineering from Keio University, Kanagawa, in 2005, 2006, and 2009. From 2009 to 2014, he was with NTT Network Service Systems Laboratories, where he was engaged in the R&D of high-speed optical communication systems, including digital coherent optical transmission systems. His research interests include high-capacity optical transport systems with ultra-wideband WDM and high-symbol rate techniques. He is a member of the Institute of Electronics, Information and Communication Engineers (IEICE) of Japan and the Institute of Electrical and Electronics Engineers (IEEE). He has served as a program sub-committee member of the Optical Communication Systems and Networks category for the 15th Pacific Rim Conference on Lasers and Electro-Optics (CLEO-PR) in 2022. He was the recipient of the Japan Society of Applied Physics Young Scientist Presentation Award in 2007 and IEICE Communications Society Optical Communication Systems (OCS) Best Paper Award in 2022.
|
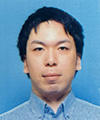 |
- Kohei Saito
- Research Engineer, NTT Network Innovation Laboratories.
He received an M.S. in electronics and applied physics from Tokyo Institute of Technology in 2013. In the same year, he joined NTT Network Service Systems Laboratories, where he was engaged in development on optical communication systems. In 2020, he moved to NTT Network Innovation Laboratories and has been investigating high-capacity optical transmission systems. He is a member of IEICE.
|
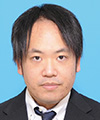 |
- Akira Masuda
- Research Engineer, NTT Network Innovation Laboratories.
He received a B.E. and M.E. in electrical engineering from Tokyo University of Science in 2011 and 2013. In 2013, he joined NTT Network Innovation Laboratories, where he was engaged in research on optical technologies for high-speed short-reach transmission. From 2019 to 2021, he was with NTT Communications Corporation, where he was engaged in the development of beyond 100-Gbit/s/ch optical WDM transmission systems. Since 2021, he has been with NTT Network Innovation Laboratories, where he is engaged in research on optical transmission technologies for high-capacity optical transport networks. He received the Young Researcher’s Award from IEICE in 2019.
|
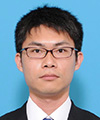 |
- Hiroki Taniguchi
- Researcher, NTT Network Innovation Laboratories.
He received a B.E. and M.E. in electrical engineering from Tokyo Institute of Technology in 2014 and 2016. In 2016, he joined NTT Network Innovation Laboratories. His research interests include photonic transport network systems. He is a member of IEICE. He was a recipient of the IEICE Communications Society OCS Young Researchers Award in 2019 and the IEICE Young Researcher’s Award in 2020.
|
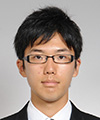 |
- Takeo Sasai
- Researcher, NTT Network Innovation Laboratories.
He received a B.E. and M.E. in electrical engineering from the University of Tokyo in 2015 and 2017. Since 2021, he has been working toward a Ph.D. in applied physics at the same university. In 2017, he joined NTT Network Innovation Laboratories. He is engaged in the modeling of optical fiber communication systems from both behavioral and data-driven approaches, particularly optical fiber nonlinearity and transceiver nonlinearity. He is a member of Optica, IEICE, and IEEE. He was a recipient of the IEICE Communications Society OCS Young Researchers Award in 2021 and the IEICE Young Researcher’s Award in 2021.
|
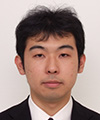 |
- Masanori Nakamura
- Research Engineer, NTT Network Innovation Laboratories.
He received a B.S. and M.S. in applied physics from Waseda University, Tokyo, in 2011 and 2013, and Ph.D. in electrical, electronic, and infocommunications engineering from Osaka University in 2021. In 2013, he joined NTT Network Innovation Laboratories, where he is engaged in research on high-capacity optical transport networks. He is a member of IEICE and IEEE. He received the IEICE Communications Society OCS Young Researchers Award in 2016 and IEICE Communications Society OCS Best Paper Award in 2022.
|
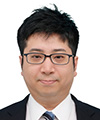 |
- Takayuki Kobayashi
- Distinguished Researcher, NTT Network Innovation Laboratories.
He received a B.E., M.E., and Dr. Eng. from Waseda University, Tokyo, in 2004, 2006, and 2019. In April 2006, he joined NTT Network Innovation Laboratories, where he was engaged in the research on high-speed and high-capacity digital coherent transmission systems. In April 2014, he moved to NTT Access Network Service Systems Laboratories and was engaged in fifth-generation mobile optical network systems. In July 2016, he moved back to NTT Network Innovation Laboratories and has been working on high-capacity optical transmission systems. His current research interests are long-haul optical transmission systems using spectrally efficient modulation formats enhanced by digital and optical signal processing. He is a member of IEICE and IEEE. He has served as a technical program committee (TPC) member of the Electrical Subsystems’ Category for the Optical Fiber Communication Conference (OFC) from 2016 to 2018. He has been serving as a TPC member of the Point-to-Point Optical Transmission Category for the European Conference on Optical Communication (ECOC) since 2018.
|
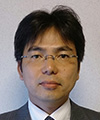 |
- Yoshiaki Kisaka
- Senior Research Engineer, Supervisor, Transport Innovation Laboratory, NTT Network Innovation Laboratories.
He received a B.E. and M.E. in physics from Ritsumeikan University, Kyoto, in 1996 and 1998. In 1998, he joined NTT Optical Network Systems Laboratories, where he was engaged in R&D on high-speed optical communication systems including 40-Gbit/s/ch WDM transmission systems and mapping/multiplexing scheme in the Optical Transport Network (OTN). From 2007 to 2010, he was with NTT Electronics Technology Corporation, where he was engaged in the development of 40/100-Gbit/s OTN framer large-scale integrated circuits. His current research interests are in high-speed and high-capacity optical transport network using digital coherent technology. From 2010, he has been contributing to the R&D of digital coherent signal processers at 100 Gbit/s and beyond. He is a member of IEICE.
|
↑ TOP
|