
You need Adobe Reader 7.0 or later in order to read PDF files on this site.
If Adobe Reader is not installed on your computer, click the button below and go to the download site.
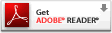
|
Regular Articles
Vol. 16, No. 2, pp. 54–57, Feb. 2018. https://doi.org/10.53829/ntr201802ra1
Electrical Current Generation by Sorting Thermal Noise
Kensaku Chida, Katsuhiko Nishiguchi,
and Akira Fujiwara
Abstract
We have achieved the operation of Maxwell’s demon with an electrical device, which is a feedback operation based on single-electron motion. Maxwell’s demon is related to the lower bound of energy consumption in electrical devices and power generation efficiency in small systems. We therefore anticipate that this achievement will contribute to creating nanoscale energy-efficient electrical devices.
Keywords: thermal noise, silicon transistor, Maxwell’s demon
1. Second law of thermodynamics and Maxwell’s demon
The second law of thermodynamics states that every physical system eventually becomes random. This law prohibits us from creating ordered motion of electrons, or electrical current, from thermal noise unless we modify the electrons externally. Maxwell’s demon is a thought experiment created by Scottish mathematician James Clerk Maxwell to contradict the second law of thermodynamics; it is an ideal entity that can perform a feedback operation by observing objects at the level of thermal noise and can create ordered motion of electrons using energy from thermal noise without our having to modify the electrons.
Maxwell’s demon has been vigorously discussed among physicists for more than 150 years because it appears to violate the second law. The discussions have clarified that Maxwell’s demon uses information about the thermal motion of electrons, which requires energy. This means that we need a certain amount of energy to obtain the information, and we can create the same amount of energy at maximum from it.
This idea leads to the concept of information thermodynamics, in which the role of information is on the same footing as energy. Information thermodynamics reveals the lower bounds of the thermodynamic cost of information processing [1] and provides us knowledge about small heat engines such as molecular motors whose motions are driven by thermal fluctuations. Maxwell’s demon is the epitome of information thermodynamics, which is considered to be related to energy-efficient biological systems.
In this study, we succeeded in generating electrical current and power with Maxwell’s demon in one of the most natural ways: observing electron thermal motion, sorting electrons with the obtained information, and outputting the sorted electrons that had large energy.
2. Silicon nanotransistors and operation of Maxwell’s demon
In the experiment, we used a silicon nanodevice on a silicon-on-insulator wafer. In our device, a single-electron box electrostatically defined by two transistors provides doors through which electrons can enter and exit the box, and a capacitively coupled detector with single-electron sensitivity detects thermal fluctuation in the box at the single-electron level (Fig. 1) [2]. By switching the transistors on and off, we can open and close the entrance to the box and the exit from it individually. The number of electrons in the box was observed in real time at a frequency of about 14 Hz by measuring the current flowing through the detector. All the measurements were done at room temperature.
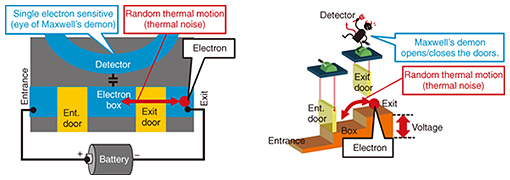
Fig. 1. Device structure. A schematic illustration of the structure of our device (left), and a corresponding schematic illustration of Maxwell’s demon (right). The nanometer-scale silicon transistors serve as doors that control the motion of electrons. Electrons move in a direction determined by the voltage as an average flow. However, at the single-electron level, the motion is random because of thermal noise. The detector can observe the random motion.
We performed feedback control based on the number of electrons in the box [3] as follows (Fig. 2). First, we open the entrance and observe random electron motion between the entrance and the box. After electrons have entered the box, we close the entrance. Then, we open the exit and observe random electron motion between the exit and the box. Finally, after the electrons have exited the box, the exit is closed. By repeating these procedures, we can move electrons from the entrance to the exit. We carefully calibrated the experimental setup to achieve the operation of Maxwell’s demon without doing any external work on the electrons [4].
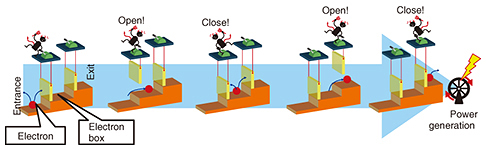
Fig. 2. Operation of Maxwell¡Çs demon. Maxwell¡Çs demon opens and closes the entrance and exit doors based on the number of electrons in the electron box, which thermally fluctuates.
3. Rectification of thermal noise with Maxwell’s demon
Carrying out the above procedures enables sorted electrons to flow as electrical current. They can even climb up the potential energy across the entrance and exit (Fig. 3); this climbing is the power generation. When the source-drain bias voltage VSD is ~30 mV, the generated power shows the maximum value of 0.5 zW (10−21 W). The quantitatively estimated information-to-energy conversion efficiency is 18%, which is reasonably high and consistent with our theoretical simulation. This consistency indicates that silicon nanodevices are an ideal platform for studying Maxwell’s demon and information thermodynamics. The simulation also demonstrated that the power output increases as the detector becomes faster and the box becomes smaller. Therefore, further advances in transistor technology will lead to an increase in the demon’s power output.
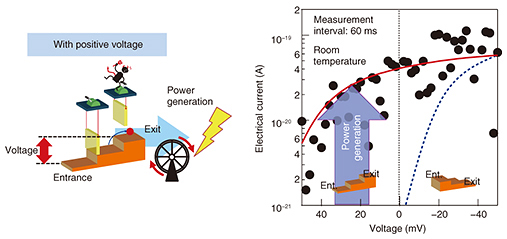
Fig. 3. Power generation with Maxwell’s demon. On the left is a schematic illustration of power generation with Maxwell’s demon. Maxwell’s demon sorts electrons with large energy and outputs them. The energy can be utilized as electrical power as depicted. The right graph shows the electrical current (in the direction corresponding to electron motion from the entrance to the exit) as a function of the voltage. The voltage represents the height of the exit relative to that of the entrance (when the exit is higher than the entrance, the voltage is positive). The dotted blue line is the expected electrical current without Maxwell’s demon. In this situation, electrons flow from the entrance to the exit only when the voltage is negative. In contrast, with Maxwell’s demon, electrons are expected to flow from the entrance to the exit even when the voltage is positive, which is shown by the red line. The experimentally obtained electrical currents, plotted as black circles, are similar to the expected value with Maxwell’s demon. When the voltage is positive, electrons climb up the voltage, and Maxwell’s demon generates electrical power as illustrated on the right.
4. Future work
The results in this work are closely related to the lower bound of energy consumption in electrical devices and the efficiency of small heat engines. To achieve high energy-to-power conversion efficiency, biomolecules, for example, molecular motors, are thought to use information about themselves to perform their operation at proper timings. Such an efficient process in biomolecules can be modeled and analyzed in the framework of Maxwell’s demon. We will attempt in the future to deepen our understanding of the mechanism responsible for the high efficiency in biological systems and thereby make an electrical device that mimics their high efficiency.
References
[1] | R. Landauer, “Irreversibility and Heat Generation in the Computing Process,” IBM J. Res. Dev., Vol. 5, No. 3, pp. 183–191, 1961. |
[2] | K. Nishiguchi, Y. Ono, and A. Fujiwara, “Single-electron Thermal Noise,” Nanotechnology, Vol. 25, No. 27, 275201, 2014. |
[3] | K. Chida, K. Nishiguchi, G. Yamahata, H. Tanaka, and A. Fujiwara, “Thermal-noise Suppression in Nano-scale Si Field-effect Transistors by Feedback Control Based on Single-electron Detection,” Appl. Phys. Lett., Vol. 107, 073110, 2015. |
[4] | K. Chida, S. Desai, K. Nishiguchi, and A. Fujiwara, “Power Generator Driven by Maxwell’s Demon,” Nat. Commun., Vol. 8, 15301, 2017. |
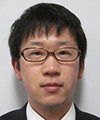 |
- Kensaku Chida
- Research Scientist, Nanodevices Research Group, Physical Science Laboratory, NTT Basic Research Laboratories.
He received a B.S. in environmental science from Okayama University in 2008 and an M.S. and Ph.D. in chemistry from Kyoto University in 2010 and 2013. He joined NTT Basic Research Laboratories in 2013. Since then, his research interests have focused on feedback control of fluctuations of single electrons in nanometer-scale devices. He is a member of the Physical Society of Japan and the Japan Society of Applied Physics (JSAP).
|
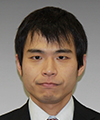 |
- Katsuhiko Nishiguchi
- Senior Research Scientist, Distinguished Researcher, Nanodevices Research Group, Physical Science Laboratory, NTT Basic Research Laboratories.
He received a B.E., M.E., and Ph.D. in electrical engineering from the Tokyo Institute of Technology in 1998, 2000, and 2002. He joined NTT Basic Research Laboratories in 2002. Since then, he has been engaged in research on the physics and technology of silicon nanometer-scale devices for large-scale integrated circuit (LSI) applications with low power consumption and new functions. He was an invited researcher at the National Center for Scientific Research in France in September 2008 and also a guest researcher at Delft University of Technology, Delft, the Netherlands, during 2012–2013. He was appointed a Distinguished Researcher of NTT in 2011. He received the IUPAP Young Author Best Paper Award at the International Conference on Physics of Semiconductors in 2000, the Graduate Student Silver Award at the Materials Research Society 2000 Fall Meeting, the Young Scientist Award at the JSAP Spring Meeting in 2000, the JSAP Outstanding Paper Award in 2013, and the Commendation for Science and Technology from the Minister of Education, Culture, Sports, Science and Technology of Japan (the Young Scientists’ Prize) in 2013. He is a member of JSAP and the Institute of Electrical and Electronics Engineers (IEEE).
|
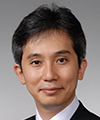 |
- Akira Fujiwara
- Senior Distinguished Researcher, Senior Manager of Physical Science Laboratory, and Group Leader of Nanodevices Research Group, NTT Basic Research Laboratories.
He received a B.E., M.E., and Ph.D. in applied physics from the University of Tokyo in 1989, 1991, and 1994. He joined NTT LSI Laboratories in 1994 and moved to NTT Basic Research Laboratories in 1996. Since 1994, he has been researching silicon nanostructures and their application to single-electron devices. He was a guest researcher at the National Institute of Standards and Technology, Gaithersburg, MD, USA, during 2003–2004. He was a director of JSAP in 2010 and 2011 and a visiting professor at Hokkaido University in 2013. He was appointed a Distinguished Researcher of NTT in 2007 and Senior Distinguished Researcher of NTT in 2015. He received the SSDM Young Researcher Award in 1998, an SSDM Paper Award in 1999 at the International Conferences on Solid State Devices and Materials, and Japanese Journal of Applied Physics Paper Awards in 2003, 2006, and 2013. He was awarded the Prize for Science and Technology from the Minister of Education, Culture, Sports, Science and Technology in 2017. He was supported by the funding program for Next Generation World-Leading Researchers (NEXT Program), the Japan Society for the Promotion of Science, in 2011–2014. He is a member of JSAP and a senior member of IEEE.
|
↑ TOP
|