
You need Adobe Reader 7.0 or later in order to read PDF files on this site.
If Adobe Reader is not installed on your computer, click the button below and go to the download site.
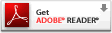
|
Regular Articles
Vol. 19, No. 11, pp. 59–64, Nov. 2021. https://doi.org/10.53829/ntr202111ra2
Development of Beyond 100G Optical Cross Connect (B100G-OXC) System
Takeshi Kawasaki, Takeshi Seki, Ken Ito,
Yasutaka Sugano, Hiroki Date, Hiroki Kawahara,
Daisaku Shimazaki, and Hideki Maeda
Abstract
The rapid increase in Internet traffic is expected due to the spread of network services such as 5G (fifth-generation mobile communication), Internet of Things, and cloud. To economically expand the capacity in the core network of the NTT Group, NTT Network Innovation Center developed the Beyond 100G Optical Cross Connect (B100G-OXC) system, which enables beyond 100-Gbit/s per wavelength optical transmission. This article gives an overview of this system.
Keywords: optical transport, DWDM, multi-level modulation
1. Capacity enhancement in the core network
NTT has expanded the capacity of optical transport systems to build both an efficient and economical optical transport network to address the constant increase in communication traffic in the core network, as shown in Fig. 1. In the current optical transport system, which has been deployed, we achieved a maximum of 8-Tbit/s capacity by multiplexing 80 channels of an optical signal with 100-Gbit/s per wavelength through the use of digital coherent technology*1. To further enhance system capacity, we developed the Beyond 100G Optical Cross Connect (B100G-OXC) system as a higher-capacity optical transport system by increasing the signal speed.
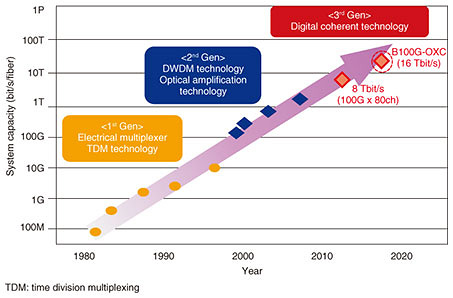
Fig. 1. Development history of optical transport systems at NTT.
*1 |
Digital coherent technology: The technology that improves both sensitivity and spectral efficiency by using a coherent detection scheme with which an optical signal is treated as a wave. It also provides high-speed signal processing that can compensate for the distortion of waveforms when transmitting through optical fiber. |
2. Technical features of B100G-OXC system
The system configuration of the B100G-OXC system is shown in Fig. 2. It is an optical transport system based on dense wavelength-division multiplexing (DWDM) and digital coherent technology (DWDM is the technology to transmit multiple wavelengths in a single fiber).
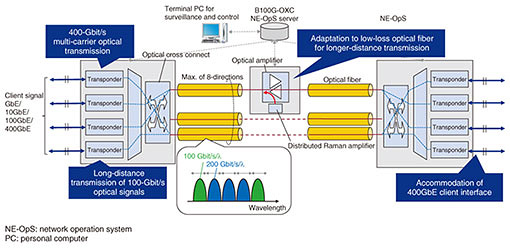
Fig. 2. Overview of B100G-OXC system.
The B100G-OXC system is also called ROADM (reconfigurable optical add/drop multiplexer) since it can configure optical nodes as “add,” “drop,” or “through” for any arbitrary optical path in a maximum of eight directions. The main technical features of the B100G-OXC system are as follows:
- 400-Gbit/s multi-carrier optical transmission
- Long-distance transmission of 100-Gbit/s optical signals
- Adaptation to low-loss optical fiber for longer-distance transmission
- Accommodation of the 400 Gigabit Ethernet (GbE) client interface
2.1 400-Gbit/s multi-carrier optical transmission
There are several ways to enhance capacity. One is to increase the number of wavelengths for multiplexing, and the other is to increase the signal speed of the optical channel per wavelength. In addition, there are mainly three ways to increase the signal speed of an optical channel [1]:
1. Increase the rate of modulation
2. Increase the degree of the multiple modulation/demodulation scheme
3. Enable multi-carrier optical transmission
With the B100G-OXC system, we increased the transmission rate per wavelength from 100 to 200 Gbit/s by changing the modulation scheme from dual polarization-quadrature phase shift keying (DP-QPSK), which is used in the conventional 100-Gbit/s-based optical system, to DP-16 quadrature amplitude modulation (QAM), maintaining the baud rate at 32 Gbaud, which is that of the conventional system (Table 1). We also achieved maximum 500-km transmission at a 400-Gbit/s data rate with no regeneration by using 2 x 200-Gbit/s optical signals.
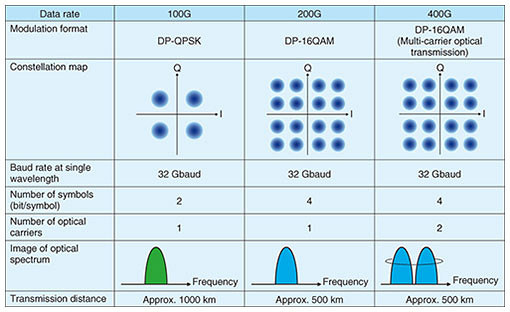
Table 1. Modulation scheme for the B100G-OXC system.
2.2 Long-distance transmission of 100-Gbit/s optical signals
In the core network, long-distance transmission is as critical as capacity enhancement in terms of building a network more economically. There is generally a trade-off between increasing the transmission rate (higher-capacity transmission) and transmission distance. Although we can achieve a higher rate of transmission by increasing the degree of the multi-level modulation scheme (for example, from DP-QPSK to DP-16QAM), transmission distance can be decreased. In the B100G-OXC system, we adopted DP-QPSK, which is the same modulation scheme as in the conventional 100-Gbit/s-based optical transport system to achieve 1000-km transmission without regeneration, which is almost double that of the conventional system. The B100G-OXC system also enables us to change the mode of the modulation scheme as well as transmission rate through software, so it is possible for us to select the optimum type of modulation more flexibly on the basis of the target distance of the route.
2.3 Adaptation to low-loss optical fiber for longer-distance transmission
We attribute 400-Gbit/s multi-carrier 500-km transmission to the fact that we adapted the B100G-OXC system to the new cutoff shifted fiber (CSF, complied with ITU-T*2 G.654.E) as well as single mode fiber (SMF, compliant with ITU-T G.652) or dispersion shifted fiber (DSF, compliant with ITU-T G.653). CSF offers a lower loss profile than that of the other types of fibers for longer transmission. It also secures a larger effective area*3 by expanding the diameter of the optical fiber core, which allows us to suppress the degradation of optical signals due to nonlinear effects that can occur during the transmission through the fiber. As a result, we can achieve longer transmission distance. The general optical characteristics of SMF, DSF, and CSF are listed in Table 2 [2].
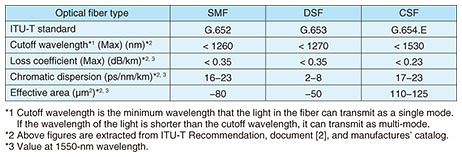
Table 2. Types of optical fibers and their characteristics.
We also achieved high-power distributed Raman amplification (DRA) in the B100G-OXC system as a key technology for longer-distance transmission, as shown in Fig. 3. DRA amplifies optical signals by using optical fiber as an amplified medium, so its gain characteristic depends on the fiber characteristics. Initially lower gain of DRA was assumed for CSF since it has a relatively larger core than those of other fibers. We then achieved high-power DRA to obtain sufficient gain even under CSF. Safety design is also important for long-term operation because we need to handle a high-power excitation light source, which has around 100 mW of output power. With the B100G-OXC system, we determined the optimum condition for the excitation light source for DRA to achieve maximum transmission performance while maintaining safety by complying with IEC*4 60825-2, which is the international standard on the safety of optical-fiber communication systems.
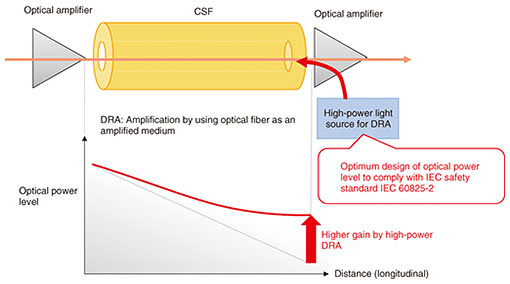
Fig. 3. High-power DRA.
2.4 Accommodation of 400GbE client interface
In the B100G-OXC system, we achieved the accommodation of the 400GbE*5 client interface in combination with 400-Gbit/s multi-carrier optical transmission. This allows us to connect with new large-scale routers or Layer 2 switches. The 400GbE interface has been standardized and is now widely used mainly in datacenters. Figure 4 shows a schematic of how 400GbE is transmitted. The incoming 400GbE signal is mapped onto 4 x OTUC*6 frames in the framer and signal processing unit inside the transponder (transceiver). Each OTUC frame has a 100-Gbit/s bit rate. Two OTUC frames are modulated in a single optical carrier, which has a 200-Gbit/s rate, then 400GbE data are transmitted using 2 x 200-Gbit/s optical carriers [3].
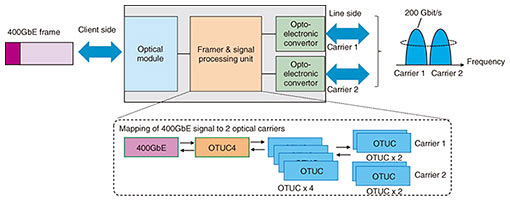
Fig. 4. Multi-carrier transmission of 400GbE signals.
*2 |
ITU-T: The Telecommunication Standardization Sector of International Telecommunication Union |
*3 |
Effective area: Indicator of how the optical power is distributed around the core in the fiber. The larger its indicator, the weaker the nonlinear effect that occurs in the fiber during transmission since the optical-power density becomes lower. |
*4 |
IEC: International Electrotechnical Commission |
*5 |
400GbE: The new specification for the high-speed Ethernet client interface standardized as international standard (Institute of Electrical and Electronics Engineers (IEEE) 802.3bs). The data-transport speed is 400 Gbit/s, which is quadruple that of 100GbE (compliant with IEEE 802.3ba). |
*6 |
OTUC: A 100-Gbit/s element (slice) of an optical channel transport unit-Cn (OTUCn). The index Cn denotes n × 100G, where n is a positive integer and C means 100G. |
3. Conclusion and future perspectives
We achieved both high-capacity and longer-distance transmission to build a core transport network more economically. NTT is now researching and developing the All-Photonics Network [4], which is one of the innovative optical transport platforms to implement IOWN (Innovative Optical and Wireless Network). We are aiming to further increase the speed of optical signals per wavelength such as 400 Gbit/s or 1 Tbit/s. We will also target furthering high-capacity transmission by using multi-band transmission [5] to expand the transmission-wavelength range or using space-division multiplexing technology such as multi-core fibers [6]. We will continue to work on further improving optical transport systems and expanding transmission capacity.
References
[1] |
H. Maeda, K. Saito, T. Kotanigawa, S. Yamamoto, F. Hamaoka, M. Yoshida, M. Suzuki, and T. Seki, “Field Trial of 400 Gbps Transmission Using Advanced Digital Coherent Technologies,” J. Lightw. Technol., Vol. 35, No. 12, pp. 2494–2499, June 2017. |
[2] |
K. Kikuchi, “Optical Information Network,” Ohmsha, 2002 (in Japanese). |
[3] |
K. Shintaku, S. Kuwahara, and S. Kobayashi, “Trends in Standardization of Mapping and Multiplexing Technologies for Optical Transport Networks,” NTT Technical Review, Vol. 18, No. 12, pp. 103–108, 2020.
https://www.ntt-review.jp/archive/ntttechnical.php?contents=ntr202012gls.html |
[4] |
A. Itoh, “Initiatives Concerning All-Photonics-Network-related Technologies Based on IOWN,” NTT Technical Review, Vol. 18, No. 5, pp. 11–13, 2020.
https://www.ntt-review.jp/archive/ntttechnical.php?contents=ntr202005fa1.html |
[5] |
T. Seki, H. Kawahara, T. Miyamura, H. Maeda, K. Hara, S. Kaneko, and J. Kani, “Evolution of Transmission Technology toward the Realization of All-photonics Network,” J. IEICE, Vol. 104, No. 5, pp. 463–469, 2021 (in Japanese). |
[6] |
T. Sakamoto, T. Mori, T. Matsui, T. Yamamoto, and K. Nakajima, “Research and Development of Next Generation Optical Fiber Using Multiple Spatial Channel,” NTT Technical Review, Vol. 15, No. 6, 2017.
https://www.ntt-review.jp/archive/ntttechnical.php?contents=ntr201706fa5.html |
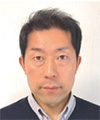 |
- Takeshi Kawasaki
- Senior Research Engineer, Transport System Project, NTT Network Innovation Center.
He received a B.S. in physics from Keio University, Tokyo, in 2001 and M.A. in arts and sciences from the University of Tokyo in 2003. He joined NTT Network Service Systems Laboratories in 2003 and studied optical transmission systems. From 2011 to 2014, he was involved in international submarine cable projects at NTT Communications. He also worked on the development project for optical transport systems and the development project for the Beyond 100G Optical Cross Connect system at NTT Network Innovation Center.
|
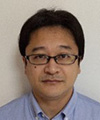 |
- Takeshi Seki
- Senior Research Engineer, Transport Network Innovation Project, NTT Network Service Systems Laboratories.
He received a B.S. and M.S. in electronics and applied physics from Tokyo Institute of Technology in 2002 and 2004. In 2004, he joined NTT Network Service Systems Laboratories, where he was engaged in research on optical cross-connect systems. He is currently developing wavelength division multiplexing (WDM) transmission systems. He is a member of the Institute of Electronics, Information and Communication Engineers (IEICE).
|
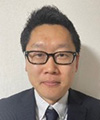 |
- Ken Ito
- Senior Research Engineer, Transport System Project, NTT Network Innovation Center.
He received a B.E. and M.E. from Waseda University, Tokyo, in 2005 and 2007. He joined NTT Network Service Systems Laboratories in 2007, where he was engaged in the research and development (R&D) of transport network systems. He is currently developing 400-Gbit/s packet transport systems. He is a member of IEICE.
|
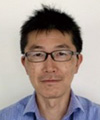 |
- Yasutaka Sugano
- Senior Research Engineer, Transport System Project, NTT Network Innovation Center.
He received a B.E. from Tokyo Denki University in 1995. He joined NTT Communications in 2003, where he was engaged in the development of the wide area Ethernet system. In 2019, he moved to NTT Network Service Systems Laboratories, where he has been engaged in the development project for beyond 100-Gbit/s packet transport systems.
|
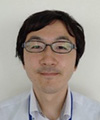 |
- Hiroki Date
- Senior Research Engineer, Transport System Project, NTT Network Innovation Center.
He received a B.E. and M.E. from Tokyo Institute of Technology in 2006 and 2008. He joined NTT Network Service Systems Laboratories in 2008, where he was engaged in the R&D of transport network systems and centralized control of IP and optical transport networks. From 2016 to 2020, he was in charge of deploying new optical transport network systems at NTT Communications. He is currently engaged in the R&D of optical cross-connect systems with advanced maintenance and operation functions.
|
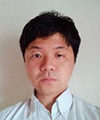 |
- Hiroki Kawahara
- Engineer, Division of Infrastructure Design, Department of Platform Services, NTT Communications Corporation.
He received a B.E. and M.E. in electrical, electronics, and information engineering from Osaka University in 2009 and 2011. He joined NTT Network Innovation Laboratories and NTT Network Service Systems Laboratories in 2011 and again in 2017, where he was involved in the R&D of large-capacity optical cross-connect and transmission systems. He is currently with NTT Communications. He is a member of IEICE.
|
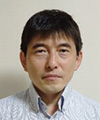 |
- Daisaku Shimazaki
- Senior Research Engineer, Supervisor, Transport System Project, NTT Network Innovation Center.
He received a B.S. in applied chemistry and M.S. in materials science from Keio University, Kanagawa, in 1999 and 2001. He joined NTT Network Service Systems Laboratories in 2001, where he researched and developed multi-layer network virtualization as a research engineer. He has been in his current position since January 2014 and is engaged in the development project for the Beyond 100G Optical Cross Connect system at NTT Network Innovation Center. He is a member of IEICE.
|
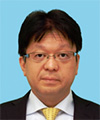 |
- Hideki Maeda
- Group Leader, Transport Network Systems Project, NTT Network Innovation Center.
He received a B.S. and M.S. in electrical engineering from Tokyo University of Science in 1992 and 1994, and received a Ph.D. from Hokkaido University in 2020, where he researched and developed long-haul large-capacity transmission systems. He was the recipient of the TELECOM System Technology Award from the Telecommunication Advancement Foundation in 2019. Dr. Maeda is a senior member of IEICE.
|
↑ TOP
|